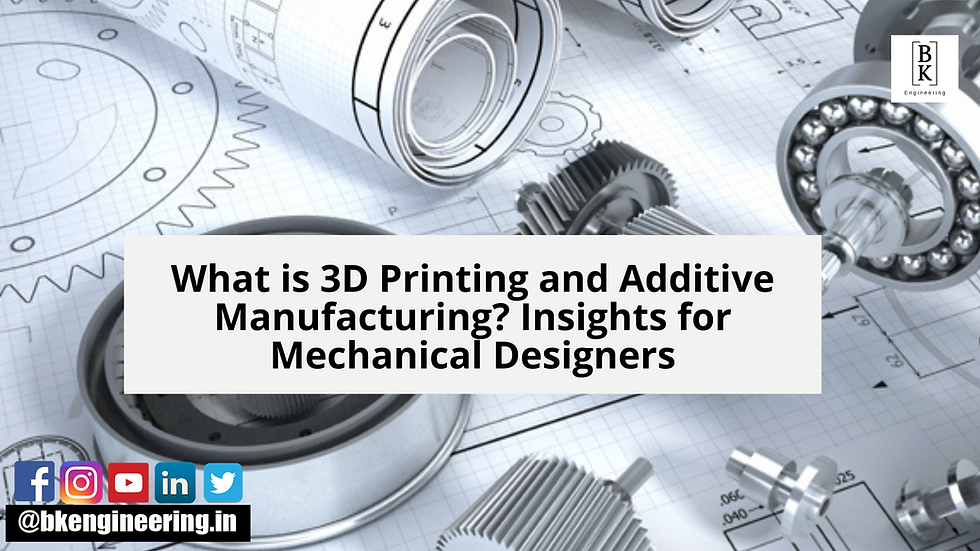
Introduction to 3D Printing
3D printing, also known as additive manufacturing, refers to processes used to synthesize a three-dimensional object in which layers of material are formed under computer control to create an object. 3D printing is an additive process, where successive layers of materials are laid down in different shapes.
The technology was first invented in the 1980s, but has seen massive growth and improvement in recent years. As 3D printers have become more accessible and affordable, more industries are adopting the technology. The key benefits of 3D printing include:
Rapid prototyping - 3D printing accelerates the process of prototyping new designs and concepts. Parts can be printed in hours and iterated upon quickly.
Customization - 3D printing makes it economical to create highly customized and personalized products tailored to individuals.
Complex geometries - The additive nature of 3D printing enables the creation of complex shapes and intricate designs not possible with traditional manufacturing methods.
Less waste - 3D printing deposits material only where needed reducing waste. Conventional manufacturing often has high material waste from milling and cutting.
Light weighting - 3D printed parts can be topology optimized and designed to be lightweight while still meeting strength needs. This is ideal for industries like aerospace.
Assembly consolidation - 3D printing enables consolidating sub-assemblies into single parts, improving reliability and reducing part counts.
The unique capabilities of 3D printing are allowing engineers to manufacture objects previously not possible. As the quality and choice of materials expand, adoption of 3D printing will continue growing across industries like aerospace, automotive, healthcare and consumer products.
Benefits of 3D Printing for Mechanical Design
3D printing offers numerous benefits for mechanical designers compared to traditional manufacturing methods. Some of the key advantages include:
Faster Prototyping
3D printing enables rapid prototyping and iteration. Designers can quickly print design concepts and test them, then make tweaks and print again. This accelerates the product development cycle. Rather than waiting weeks for machine shop prototypes, 3D printed parts can be produced in hours or days.
Customization
3D printing makes it economical to create fully customized parts tailored to specific applications or customers. Mechanical components can be optimized for the exact loads, fit, and operating environment needed.
Complex Geometries
The additive nature of 3D printing allows complex geometries like lattices, internal voids, and organic shapes that cannot be made with subtractive methods. This geometric freedom benefits areas like light weighting.
Lightweight Parts
3D printing facilitates topology optimization and generative design techniques that create highly organic, skeletal structures optimized for strength and stiffness at the lowest possible weight. Light weighting parts this way was not feasible before.
3D Printing Materials
3D printing can utilize a wide range of materials including plastics, metals, concrete, food, and even living tissues. Each material has advantages and limitations that need to be considered when designing parts for 3D printing.
Plastics
The most common materials in 3D printing are plastics like ABS, PLA, PETG, and nylon. These thermoplastics can produce strong functional parts with good accuracy and surface finishes. PLA and PETG are popular for their low melting points while ABS and nylon offer higher thermal resistance. The variety of available plastics allows designers to choose the best material properties for an application.
Metals
Metal 3D printing methods like selective laser sintering, direct metal laser sintering, and binder jetting can create complex metal parts using steel, aluminum, titanium, nickel alloys, and more. The high strength and thermal resistance of metal 3D printed parts make them suitable for functional components, tooling, and end-use production applications. However metals can be expensive and have limitations on geometric complexity.
Concrete
3D printing of concrete uses a mortar or binder material to build structural components in layers. This allows fast, economical construction of custom concrete shapes. While low in resolution compared to other materials, concrete printing enables architects and builders to experiment with new structures and geometries.
Food
Food safe materials are used to 3D print confections, snacks, or components that can be cooked. This allows unique customizable shapes and artful food designs. Edible 3D printing brings new possibilities for specialty cuisine.
Tissues
3D bioprinting uses bio-inks containing living cells to print functional human tissue and organs. This emerging field promises future medical breakthroughs, but currently faces challenges in vascularization and manufacturing scale.
By understanding the capabilities and tradeoffs of each 3D printing material, mechanical designers can select optimal options for their applications and take advantage of 3D printing's unique geometric freedom. The range of materials is rapidly expanding, bringing new potential benefits in cost, customization, and performance.
3D Printing Technologies
Fused Deposition Modeling (FDM)
Fused deposition modeling (FDM) is one of the most common and affordable 3D printing technologies. It works by heating and extruding thermoplastic filament, depositing it layer by layer to create a 3D object.
Advantages:
Low cost of both printers and materials
Reliable and easy to use
Good strength and thermal properties for functional parts
Variety of material options including ABS, PLA, nylon, PC, PETG
Disadvantages:
Lower resolution and accuracy compared to other technologies
Visible layer lines on angled and curved surfaces
Limited overhangs and bridges without support material
Warping can be an issue with some materials
Overall, FDM is a great choice for quickly and affordably printing mechanical concept models, functional prototypes, production tools and end-use parts. The accuracy may not be suitable for intricate details.
Selective Laser Sintering (SLS)
Selective laser sintering (SLS) uses a laser to fuse powdered material, layer by layer. The powder surrounds the printed parts and provides support during printing.
Advantages:
Good mechanical strength with nylon materials
Does not require support structures so complex geometries are easier to print
Variety of material options including nylon, glass-filled nylon, elastomers
Allows parts to be nested within the build volume for efficiency
Disadvantages:
Porous surfaces that may require sealing for some applications
Smaller build volumes than some other technologies
Limited material options compared to other processes
Comparatively higher equipment costs
SLS can be a great process for functional prototypes or end-use parts, but the surface finish and accuracy is lower than some processes. The variety of nylon materials provide good durability.
Stereolithography (SLA)
Stereolithography (SLA) uses an ultraviolet laser to cure liquid photopolymer resin layer by layer.
Advantages:
Exceptional surface finish and part accuracy
Good mechanical properties with some resins
Allows complex geometries without supports
Quick printing speeds for small parts
Disadvantages:
Limited material options compared to other processes
Comparatively smaller build volumes
Resin can be messy to work with
May require additional post-processing and curing
SLA is one of the best processes when superior surface finish, detail resolution, and accuracy is critical. It excels at small, complex parts. The material options are relatively limited compared to other processes.
Design Considerations
With 3D printing, mechanical designers need to think differently about part creation compared to traditional manufacturing methods. Some key design considerations include:
Design for Additive Manufacturing
Leverage complex geometries like lattices, internal channels, and organic shapes that can only be produced through 3D printing.
Optimize parts for weight reduction through generative design algorithms and topology optimization.
Consolidate assemblies into single parts by integrating fasteners, hinges, and other components.
Create lightweight parts with strength exactly where needed by using unique infill patterns.
Understand Capabilities and Limitations
Consider minimum feature size, accuracy, surface finish, and other capabilities of the 3D printing process.
Design parts according to the size limitations of the 3D printer build volume.
Account for any anisotropic properties and directional weaknesses in 3D printed parts.
Understand how overhangs, bridges, and angles affect printability.
Plan for Post-Processing
Design parts with post-processing in mind. Include access holes for powder removal and surface treatments.
Account for shrinkage, warpage, and changes in dimensions after printing.
Determine if support structures are needed and how they will be removed after printing.
Decide how parts will be finished - whether sanding, painting, coating, or other methods.
Industry Adoption of 3D Printing
The adoption of 3D printing by major companies is rapidly increasing as the technology matures and more use cases emerge. Companies across industries are investing heavily in 3D printing and integrating it into their design and production workflows.
Investments by Major Companies
Many Fortune 500 manufacturers have invested millions in 3D printing, seeing its potential to transform their business. Boeing has used 3D printing to create over 20,000 parts for 10 different aircraft platforms. Airbus has a division dedicated to 3D printing and uses it to produce cabin parts. General Electric utilizes 3D printing to produce fuel nozzles for jet engines. Other major companies investing in 3D printing include Ford, Johnson & Johnson, Nokia, Under Armour, and more.
Production Use Cases
3D printing is going beyond prototyping and being used for end-use production parts across sectors like aerospace, automotive, medical, consumer products, industrial machinery, and more. For example, hearing aid manufacturers like Phonak and Starkey produce millions of custom 3D printed hearing aid shells each year. Align Technology uses 3D printing to make millions of clear orthodontic aligners annually. 3D printing enables mass customization and on-demand manufacturing for companies.
Strategic Partnerships
To accelerate 3D printing adoption, many large manufacturers are entering strategic partnerships with 3D printing companies. For example, Lockheed Martin and Arconic have partnered with 3D printing startups like Relativity Space and Desktop Metal. Audi has partnered with equipment maker EOS to develop processes for 3D printing aluminum parts. Partnerships like these enable companies to fast-track development of new 3D printing technologies and integrate them into production. They facilitate the transfer of 3D printing from labs to shop floors.
Applications Transforming Industries
3D printing is enabling breakthroughs in industries like aerospace, automotive, medical devices, food, and construction.
Aerospace
Aerospace companies are using 3D printing to create lightweight parts that are optimized for flight. Complex geometries like airfoil blades can be printed as a single piece, improving performance. NASA is pushing the limits of 3D printing in space, installing printers on the International Space Station to create tools and spare parts on demand.
Automotive
Automakers have been early adopters of 3D printing for rapid prototyping of new models. Now they are using the technology to manufacture custom lightweight parts, jigs and fixtures for assembly lines, and personalized accessories or options for customers. 3D printing improves flexibility and enables faster iteration.
Medical Devices
3D printing has revolutionized medical devices by enabling personalized design matched to a patient's unique anatomy. Surgeons can replicate the exact shape needed for implants, prosthetics, or anatomical models from medical scan data. The FDA has approved several 3D printed medical devices including cranial implants and hip joints. More customized, patient-specific equipment is on the horizon.
Custom Prosthetics
For prosthetic limbs, 3D printing makes customized fit easier and more affordable. The geometry and size can be tailored to match the exact limb measurements rapidly without expensive tooling. Children who quickly outgrow prosthetics get an improved experience with 3D printing.
Food
3D food printers produce edible decorations and complex pastry shapes that are impossible by hand. Research is underway into customized 3D printed nutrition and texture for the elderly. The technology may someday enable personalized meals with ideal calories, vitamins, and tastes for each individual.
Buildings
In architecture, 3D printing builds structures, wall panels, and architectural models. The world's first 3D printed homes and offices are being built with concrete printed by massive robotic arms. 3D printing may allow for novel organic shapes only limited by the imagination of architects.
Skills Needed for 3D Printing Engineers
To be effective in the role of a 3D printing engineer, certain specialized skills are required beyond a traditional mechanical engineering background.
3D Modeling Expertise
Expert skills in CAD software tools like SolidWorks, Autodesk Inventor, or CATIA for designing 3D models intended for printing are essential. The designer needs an acute understanding of how the model geometry will translate to a print-ready file. Designing models specifically for additive manufacturing requires a different approach than for traditional manufacturing methods.
3D Printing Software Knowledge
Knowledge of common software tools for 3D printing file preparation like Ultimaker Cura, Simplify3D or KISSlicer is required. This includes properly configuring print settings like layer height, infill, supports, and print speed for optimal results. The ability to diagnose and correct issues with 3D print files is also important.
Material Science Expertise
Understanding the properties and behaviors of various materials used in 3D printing like plastics, resins, metals, concrete, etc. allows for selecting the proper material for an application. Materials need to be paired with the right printing process and tuned to the specific part requirements.
Testing and Analysis Proficiency
Mechanical testing skills including destructive and non-destructive testing allows assessment of 3D printed part quality. This is key to ensuring prints meet design specifications before final production. Understanding how to optimize designs based on testing data is also an important capability.
Challenges With 3D Printing for Mechanical Design
3D printing can offer many benefits, however it also comes with some unique challenges. When evaluating the technology for mechanical applications, designers should consider the following challenges:
Cost
The printers, materials, and post-processing involved in 3D printing can be quite expensive compared to traditional manufacturing methods for mass production. The cost per part is higher for lower volume production runs. For prototyping and custom applications the costs may be justified, but higher volume production is often still cheaper with techniques like plastic injection molding.
Speed
Most 3D printers are still slow compared to high-speed production lines for manufacturing. Cycle times for 3D printing can range from hours to over a day depending on the technology, materials, and part size. This limits 3D printing for higher volume applications where speed is important.
Accuracy and Tolerances
Most 3D printers have limited resolution accuracy in the range of 0.100-0.500mm. It can be difficult getting accurate prints with tight tolerances unless using industrial grade 3D printers. Some post-processing may be required to achieve the desired accuracy.
Material Properties
While materials are improving, 3D printed plastics still cannot match the performance of traditionally injection molded plastics. The layering process creates weak points and anisotropy. Some materials like metals achieve comparable properties, but have other tradeoffs. Material choice is limited compared to other processes.
Design Limitations
3D printers cannot produce certain designs like small enclosed voids. Designs must be optimized for layer-based printing with supports. Not all geometries are feasible. Designers also have to take into account orientation, build volume constraints, etc.
3D printing comes with tradeoffs compared to conventional manufacturing. Mechanical designers should take these challenges into account when determining if 3D printing is the right choice for an application. Improvements in printers and materials continue to make 3D printing more viable for a wider range of uses.
The Future of 3D Printing and Additive Manufacturing
3D printing technology is advancing rapidly, opening up new possibilities in mechanical engineering and manufacturing. Here are some key developments to expect in the future:
Faster Printing
Hardware and software improvements will enable 3D printers to run much faster. Print times that take hours or days now will be reduced to minutes or hours.
High-speed sintering printers like HP's Multi Jet Fusion can already print production-quality parts up to 10x faster than older methods. These speeds will improve further.
Continuous liquid interface production (CLIP) reduces print times by constantly pulling the printed part out of a pool of photopolymer resin. This eliminates the wait time between layers.
New Materials
More high-performance materials like carbon fiber, fiberglass, and metal alloys will become printable on desktop 3D printers.
Multi-material printing will allow parts to be printed using different materials for different components or regions.
Bioprinting will enable 3D printing of living tissues using "bio-inks" made from human cells. This could transform medicine.
Generative Design
Algorithms can produce optimized organic 3D models tailored for 3D printing and impossible to manufacture otherwise.
Generative design software like Autodesk Within allows designers to define goals and constraints, then uses AI to generate designs.
This will lead to lighter, stronger mechanical parts that resemble bone or tree structures.
Mainstream Adoption
3D printing is expected to become a mainstream manufacturing method used for low- to mid-volume production.
The global 3D printing market is predicted to grow from $12 billion in 2019 to over $34 billion by 2024.
3D printing will allow faster, cheaper, customized production of mechanical parts on demand.
In summary, 3D printing technology will continue rapidly advancing and find widespread use for manufacturing in the years ahead. It is a transformative force that mechanical designers should embrace.
Commentaires